Health care robotics
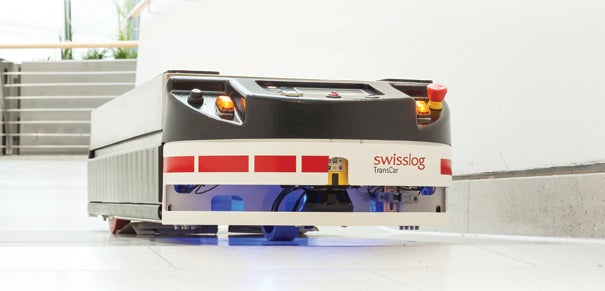
Automated guided vehicles can help hospitals take control of large-payload, on-demand and routine material transport activities.
Health care is channeling the future to present times by utilizing robotic technologies in such functions as food service, medication distribution, infection control, surgery and even diagnosing patients.
The demand is projected to grow exponentially because of their many advantages. According to proponents, robots can curtail labor costs, add operational efficiencies, increase precision, create better clinical outcomes and replace humans in potentially unsafe situations.
But these systems are not one-size-fits-all and health facilities professionals need to be part of a careful and deliberative team-based process to make the right decisions regarding selection, technology maturity, logistics, infrastructure planning and maintenance.
Factors to consider
Although most people think of surgical and interventional robots, there are many other types of robots playing important roles throughout health care facilities.
These include disinfecting patient rooms, emergency departments and operating rooms; decontaminating and sterilizing surgical instruments; preparing and dispensing medications; collecting, preparing, transporting, analyzing and storing laboratory samples; and transporting medical supplies, medications, meals, linens and trash.
There are many factors to consider before acquiring and implementing one of these systems in a health care facility. Regardless of the specific technology being considered, all key stakeholders should be identified and included at the beginning of the decision-making process.
The process should involve a thorough review of the state of the technology, regulatory approvals and requirements, departmental and interdepartmental impacts, safety measures, physician and staff training, staffing impact, infrastructure demands, service and maintenance needs, and information technology requirements.
The latter of these include interfacing; cost analyses including equipment, supplies and comparative modality costs; length-of-stay, readmissions and clinical outcomes impact; reimbursement projections; marketing; and patient and staff satisfaction and acceptance.
Robots and requirements
Nonsurgical health care robots and their respective uses, efficiencies, maintenance requirements and infrastructure components include:
Autonomous mobile transport robots
These deliver supplies, medications, meals, linens and trash throughout a hospital. Some models require dedicated hallways, floor tracks, large docking areas and navigation hardware to be installed throughout the facility.
An automated guided vehicle (AGV) does not need navigation hardware, staging depots or specific infrastructure alterations. AGVs follow a map of the facility that is created with highly accurate laser floor dimensions. The facility layout is programmed into routes, including elevators, automatic doors, delivery points and charging stations, that are loaded into the robot's memory.
AGVs also use onboard sensors to adjust to the dynamic and changing hallways for safe navigation. AGVs can use existing Wi-Fi systems to communicate with elevators, automatic doors and fire alarms. Their designs include touch screens for selecting delivery points as well as personal identification number pads and biometric fingerprint readers for security. AGVs can run for 10 hours or more at a time and automatically return to the docking station for recharging. AGV platforms also allow for the use of dining carts, linen racks, trash bins, material racks and dirty linen bins that can be pushed on the base and automatically raised by a motorized lift and transported. This prevents employee injuries from moving heavy loads and allows them to be available for other tasks.
Some vendors have a 24/7 command center that monitors the status of the AGVs in real time. If an AGV needs help, an alert is generated to the command center staff. The command center staff then can connect to it over the Internet using a secure, virtual private network connection, use the onboard sensors to assess the situation, and steer and navigate it remotely. The nonprofit ECRI Institute, Plymouth Meeting, Pa., recommends that service agreements include the availability of backup-loaner AGVs when owned or leased AGVs are down. Single AGV units can have a price range of $25,000 to $140,000, depending on options and capabilities.
Environmental disinfection robots
These have increased in popularity and are being trialed and implemented in hospitals to perform disinfecting of patient rooms and operating rooms.
Two methods for disinfection are ultraviolet (UV) light and hydrogen peroxide vapors (HPV). UV light effectively kills microorganisms, including spores, and hydrogen peroxide-based systems utilize oxidative processes to kill microorganisms, including spores. While neither method can be used in an occupied space, both are used after manual room cleaning to ensure maximum effectiveness; also both methods add to room turnover time, but leave no harmful residue.
Disinfecting UV robots are portable, automatic and self-contained. Some units, which use low-pressure mercury lamps, have a list price ranging between $104,000 and $124,500. According to ECRI Institute's proprietary price-paid database, a 36-month lease agreement for a non-mercury, xenon-lamp UV robot costs about $3,000 per month.
The hydrogen peroxide-based robots range from $44,000 to $64,000. An important safety requirement before using these robots is to seal the ducts and other room openings to prevent toxic HPV from escaping.
For both UV and HPV, the robots are remotely controlled, utilize door sensors to automatically shut down if the door in the cleaning area is opened, and include warning signs that are to be deployed before use. If a health care organization outsources environmental services, it should consider including the use of robotic disinfection systems in the contracted services.
Laboratory robots
These are used to manage specimen tubes, prepare them for testing, and allow for sample tracking throughout the testing process from collection to results.
The systems have various modules for sample preparation, transport to analytical instruments — such as hematology, chemistry and immunoassay analyzers — and post-analytical storage. Each module typically includes several units such as decappers, sorters, centrifuges, analyzers and refrigerators. The transport track system often is composed of two or more lanes, which are dedicated to various areas of the laboratory with one lane dedicated as the stat lane. The length of the track system is configurable and some can turn corners to accommodate the room size.
When the laboratory has streamlined its manual process and determined which type of system will be most beneficial, it must assess building and space requirements for the physical installation. Larger systems may require extensive remodeling and complete systems may not be adaptable enough to fit into the existing laboratory space. The floor space needed is usually greater than the actual dimensions quoted for the system because of the clearance needed behind the modules for maintenance and restocking disposables.
Location of utility hookups for water and compressed air to run robotic lines needs to be addressed; some units can alternatively hook up to separate compressed air cylinders instead of facility lines. Environmentally, these systems have processes that reduce waste, and some modules have energy-saving features such as standby or hibernation modes or automatic shutoffs. Improvements to current systems include more intelligent sample processing, better interfacing capabilities and radio-frequency identification technology to eliminate the need for multiple barcode readers.
Integration of this automated system with existing laboratory and hospital information technology (IT) is a major consideration, primarily due to the bidirectional interfaces needed to receive and transport samples and deliver results. Most of the systems are designed to interface with laboratory or hospital information systems and are very reliable.
Robotics can improve efficiency, decrease testing turnaround times, decrease the risk of a technician's exposure to biohazardous materials and decrease their risk of repetitive-stress injuries. Laboratory robots have proven to be a major cost-saving investment in large laboratories, and modular systems have made robotics cost-effective for smaller laboratories. The larger systems have been quoted between $425,000 and $1.9 million for equipment only.
Pharmaceutical robots
There are a couple of robots commonly serving the pharmaceutical areas of hospitals.
Located in the oncology infusion area or the main pharmacy, intravenous (IV) robots are used in areas of hazardous IV compounding to prepare and fill IV bags and syringes. The module managing the process of drug preparation can be interfaced with oncology or pharmacy computer systems. With this system, the pharmacist first reviews the medication order and then the IV robot receives and processes the order. The nurse picks up the completed compound and administers it to the patient.
There are a number of purported advantages for using an IV robot to compound chemotherapy and medications. It reduces operator exposure to hazardous drugs, produces precise dosing and improves productivity. Other benefits include validation of performance, integrity of high-efficiency particulate air (HEPA) filtration, decreased contamination of the preparation area, barcoding and automated waste management.
Sample specifications for IV robots include Health Level 7 and touch-screen interfaces, barcode reader, high-precision scale, powder-drug mixer, HEPA filters and automatic trash seals. An example of the physical specification requirements of one of these machines is a height of 7 feet 10 inches, a width of 6 feet 10 inches, and a depth of 5 feet 3 inches, including ventilation and air pressure requirements.
A large dispensing model may have a footprint of 31 square feet. It also will require a virtual, private-network, high-speed Internet hookup. The cost for equipment alone can range from $400,000 to $1.5 million.
Usually located in a hospital's central pharmacy department, medication-dispensing systems can be a significant investment, but can automate up to 90 percent of medication dispensing from a hospital's main pharmacy. It eliminates time-consuming manual tasks while offering accuracy, control, compliance and real-time inventory management. This reduces excess inventory and expired medications.
The large systems require significant modifications to the pharmacy layout and infrastructure. Also available are compact robotic systems that combine prescription filling, labeling, verification and dispensing of tablets, capsules and unit-of-use medication at a single work station.
Health facilities professionals should suggest a larger footprint than quoted by the manufacturer to allow clearance for maintenance, adding bulk medications and egress. Because of the significant variety of medication dispensing robots and their options, the equipment price can range from $25,000 to $4.5 million. These price points do not include installation and IT costs, which may be significant. Most of the systems are designed to interface with pharmacy or hospital information systems.
Sterile processing robots.
These devices for the central services areas of health facilities are still under various states of development and pilot testing, but hold great promise.
Among these devices is a robot located in a 5-foot square Plexiglas cubical enclosure and surrounded by stations for cleaning, sorting, inspecting, counting and repacking instruments. The cubical has a panel door that is lifted to push through the dirty instrument trays and to retrieve clean ones. One task the robot cannot do well so far is to take apart instruments that have multiple parts; however, it can do bulk work, such as prepare major orthopedic trays. This robot would represent a significant safety improvement for employees by avoiding splashing or exposure to blood-borne pathogens.
As with current central sterile decontaminators and washers, these robots have space and utility infrastructure requirements and need delineation of soiled and clean areas.
Others in the field
Other nonsurgical health care robots competing for attention in the burgeoning medical robotics field include:
- Daily care robots designed to support elderly and disabled people in such activities as preparing and serving meals and daily care tasks;
- Exoskeleton-powered robotic systems used to help paraplegic patients to stand with the hope it will reduce morbidity and augment physical therapy for patients with impaired limbs;
- Noninvasive, radiosurgery robotics that use image-guided and computer-controlled robotics to deliver beams of high-energy radiation of great precision to shrink or eradicate tumors without surgery;
- Telepresence robots suited for virtual medical consultations or medical visits where there is a long distance between patient and doctor;
- Therapeutic robots used to enhance the therapy of developmentally disabled children and record their progress.
Successful implementation
Most health care robots are costly investments and often have significant safety, infrastructure and IT considerations.
Successful implementation of robotics in a health care facility lies in thoroughly vetting the technology, comprehensive planning, well-timed and planned installations, appropriate training and periodic reviews of operational processes.
Keeping apprised of these technological developments is essential for forward-thinking health care facilities.
Debra Ann Maleski is a senior associate in the applied solutions group at ECRI Institute, Plymouth Meeting, Pa. She can be reached at dmaleski@ecri.org.
Advances in surgical and interventional robots
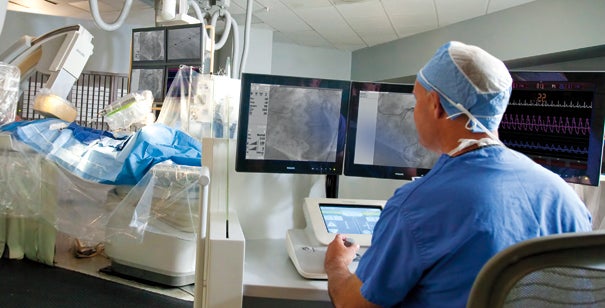
A physician performs an angioplasty with the help of a vascular robot system.
Attracting the most attention in the health care robotics field, surgical and interventional robots are designed to improve the precision of surgeries and interventional procedures, reduce complication rates and reduce costs in terms of length of stay. They include:
Surgical telemanipulation systems
Initially, these were developed to facilitate minimally invasive surgery and to assist surgeons' performing surgical procedures that otherwise would be difficult with conventional open or laparoscopic techniques. Touted clinical benefits include reduced surgical trauma to tissue, reduced blood loss and need for transfusions, tremor filtering, improved accuracy and precision, less postoperative pain and discomfort, less risk of infection, shorter hospital stay, faster recovery and return to normal activities, less scarring and improved cosmesis.
Robotic surgical systems consist of a surgeon's console, a computerized control system, a patient side cart with two or three instrument arms and an endoscope with fiber-optic camera; an optional fourth arm potentially can replace an assisting surgeon or first assistant. The systems offer 3-D, high-definition vision systems with up to 10x magnification, digital zoom, adjusted aspect ratios for viewing area, an integrated fourth robotic arm and a motorized patient cart. Proprietary instruments have articulated ends to offer surgeons better dexterity and full range of motion through small incisions. The useful life for these systems is marketed at five years, but individual components such as the console, side cart, robotic arms, 3-D vision system and instruments, can last from four to seven years.
Some systems can perform routine and systemic safety checks to ensure patient safety and reduce downtime. ECRI Institute, Plymouth Meeting, Pa., quotes capital costs in the $2 million range per system depending on the configuration and maintenance costs at more than $100,000 per year.
Acquisition cost may decrease as the technology has greater diffusion and a greater number of competitors.
Surgical-assist robot.
These allow for handling of surgical instruments at the operative site. The robot responds to voice commands from the operating surgeon, hands the surgical instruments to the surgeon with a magnetic mechanical arm, and views and recovers instruments once the surgeon places them down. These fast-developing robots are efficient, accurate and may minimize operating room labor and associated costs.
Vascular interventional robotic system
This new system brings precision, accuracy and greater safety to coronary angioplasty procedures to help optimize clinical outcomes. The system, which was designed to fit and be utilized in current existing cardiac cath labs and hybrid operating rooms, enables precise, robotic-assisted control of coronary guide wires, balloons and stent devices by the physician who is located in a radiation-shielded, ergonomic interventional cockpit or control room. Capital costs may vary from $400,000 to $474,900, depending on configuration. Acquisition considerations include training, facilities review for installation, and service and supply contracts.
Orthopedic surgical robots
Health care facilities are working closely with their orthopedic surgeons to consider adding this new technology to their high-tech portfolio. These robots assist orthopedic and neurosurgeons in total hip arthroplasty, total knee arthroplasty, partial knee resurfacing and spine implant placement.
Before investing in these devices, health facilities must assess the system to determine if it can add value and whether it is compatible with major vendor implants. Equipment costs range from $800,000 to $1.3 million, depending on configuration; and service costs can average $100,000 per year. There are no significant facility implications beyond space for storage in the operating room.